In our product programme we offer our customers two classes of Stainless Steel grades that have an excellent resistance to corrosion stainless steel. Austenitic-ferritic Duplex stainless steels are characterised by their excellent mechanical qualities, particularly their high stress corrosion cracking resistance. They are especially well-suited for maritime applications and in the chemical industry. Their excellent resistance to corrosion enables them to withstand a chloride medium, particularly under mechanical stress. This makes them superior to austenitic steel in many cases. The category of austenitic corrosion resistant stainless steel tube primarily includes materials with higher alloys (e.g. nickel, chrome and molybdenum). They are resistant to different types of corrosion caused by wet chemical influences, and are still able to maintain an austenitic face centred cubic matrix. This creates a range of highly versatile stainless steel.
Although one of the main reasons why stainless steels are used is corrosion resistance, they do in fact suffer from certain types of corrosion in some environments and care must be taken to select a grade which will be suitable for the application.Corrosion can cause a variety of problems, depending on the applications: Perforation such as of tanks and pipes, which allows leakage of fluids or gases,
Loss of strength where the cross section of structural members is reduced by corrosion, leading to a loss of strength of the structure and subsequent failure. Degradation of appearance, where corrosion products or pitting can detract from a decorative surface finish,
Finally, corrosion can produce scale or rust which can contaminate the material being handled; this particularly applies in the case of food processing equipment.
Corrosion of stainless steels can be categorised as one of:-
· General Corrosion
· Pitting Corrosion
· Crevice Corrosion
· Stress Corrosion Cracking
· Sulphide Stress Corrosion Cracking
· Intergranular Corrosion
· Galvanic Corrosion
· Contact Corrosion
General Corrosion
Corrosion whereby there is a general uniform removal of material, by dissolution, eg when stainless steel is used in chemical plant for containing strong acids. Design in this instance is based on published data to predict the life of the component.
Published data list the removal of metal over a year. Tables of resistance to various chemicals are published by various organisations and a very large collection of charts, lists, recommendations and technical papers are available though stainless steel manufacturers and suppliers.
Pitting Corrosion
Under certain conditions, particularly involving high concentrations of chlorides (such as sodium chloride in sea water), moderately high temperatures and exacerbated by low pH (ie acidic conditions), very localised corrosion can occur leading to perforation of pipes and fittings etc. This is not related to published corrosion data as it is an extremely localised and severe corrosion which can penetrate right through the cross section of the component. Grades high in chromium, and particularly molybdenum and nitrogen, are more resistant to pitting corrosion.
Pitting Resistance Equivalent number (PRE)
The Pitting Resistance Equivalent number (PRE) has been found to give a good indication of the pitting resistance of stainless steels. The PRE can be calculated as:
PRE = %Cr + 3.3 x %Mo + 16 x %N
One reason why pitting corrosion is so serious is that once a pit is initiated there is a strong tendency for it to continue to grow, even although the majority of the surrounding steel is still untouched.
The tendency for a particular steel to be attacked by pitting corrosion can be evaluated in the laboratory. A number of standard tests have been devised, the most common of which is that given in ASTM G48. A graph can be drawn giving the temperature at which pitting corrosion is likely to occur, as shown in Figure 1.
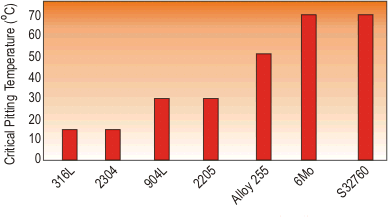
Figure 1. Temperature at which pitting corrosion is likely to occur
This is based on a standard ferric chloride laboratory test, but does predict outcomes in many service conditions.
Crevice Corrosion
The corrosion resistance of a stainless steel is dependent on the
presence of a protective oxide layer on its surface, but it is possible under certain conditions for this oxide layer to break down, for example in reducing acids, or in some types of combustion where the atmosphere is reducing. Areas where the oxide layer can break down can also sometimes be the result of the way components are designed, for example under gaskets, in sharp re-entrant corners or associated with incomplete weld penetration or overlapping surfaces. These can all form crevices which can promote corrosion. To function as a corrosion site, a crevice has to be of sufficient width to permit entry of the corrodent, but sufficiently narrow to ensure that the corrodent remains stagnant. Accordingly crevice corrosion usually occurs in gaps a few micrometres wide, and is not found in grooves or slots in which circulation of the corrodent is possible. This problem can often be overcome by paying attention to the design of the component, in particular to avoiding formation of crevices or at least keeping them as open as possible. Crevice corrosion is a very similar mechanism to pitting corrosion; alloys resistant to one are generally resistant to both. Crevice corrosion can be viewed as a more severe form of pitting corrosion as it will occur at significantly lower temperatures than does pitting.
Stress Corrosion Cracking (SCC)
Under the combined effects of stress and certain corrosive environments stainless steels can be subject to this very rapid and severe form of corrosion. The stresses must be tensile and can result from loads applied in service, or stresses set up by the type of assembly e.g. interference fits of pins in holes, or from residual stresses resulting from the method of fabrication such as cold working. The most damaging environment is a solution of chlorides in water such as sea water, particularly at elevated temperatures. As a consequence stainless steels are limited in their application for holding hot waters (above about 50°C) containing even trace amounts of chlorides (more than a few parts per million). This form of corrosion is only applicable to the austenitic group of steels and is related to the nickel content. Grade 316 is not significantly more resistant to SCC than is 304. The duplex stainless steels are much more resistant to SCC than are the austenitic grades, with grade 2205 being virtually immune at temperatures up to about 150°C, and the super duplex grades are more resistant again. The ferritic grades do not generally suffer from this problem at all.
In some instances it has been found possible to improve resistance to SCC by applying a compressive stress to the component at risk; this can be done by shot peening the surface for instance. An
other alternative is to ensure the product is free of tensile stresses by annealing as a final operation. These solutions to the problem have been successful in some cases, but need to be very carefully evaluated, as it may be very difficult to guarantee the absence of residual or applied tensile stresses.
From a practical standpoint, Grade 304 may be adequate under certain conditions. For instance, Grade 304 is being used in water containing 100 – 300 parts per million (ppm) chlorides at moderate temperatures. Trying to establish limits can be risky because wet/dry conditions can concentrate chlorides and increase the probability of stress corrosion cracking. The chloride content of seawater is about 2% (20,000 ppm). Seawater above 50°C is encountered in applications such as heat exchangers for coastal power stations.
Recently there have been a small number of instances of chloride stress corrosion failures at lower temperatures than previously thought possible. These have occurred in the warm, moist atmosphere above indoor chlorinated swimming pools where stainless steel (generally Grade 316) fixtures are often used to suspend items such as ventilation ducting. Temperatures as low as 30 to 40°C have been involved. There have also been failures due to stress corrosion at higher temperatures with chloride levels as low as 10 ppm. This very serious problem is not yet fully understood.
Sulphide Stress Corrosion Cracking (SSC
Of greatest importance to many users in the oil and gas industry is the material’s resistance to sulphide stress corrosion cracking. The mechanism of SSC has not been defined unambiguously but involves the conjoint action of chloride and hydrogen sulphide, requires the presence of a tensile stress and has a non-linear relationship with temperature.
The three main factors are Stress Level, Environment and Temperature.
Stress Level
A threshold stress can sometimes can be identified for each material – environment combination. Some published data show a continuous fall of threshold stress with increasing H2S levels. To guard against SSC NACE specification MR0175 for sulphide environments limits the common austenitic grades to 22HRC maximum hardness.
Environment
The principal agents being chloride, hydrogen sulphide and pH. There is synergism between these effects, with an apparently inhibiting effect of sulphide at high H2S levels.
Temperature
With increasing temperature, the contribution of chloride increases but the effect of hydrogen decreases due to its increased mobility in the ferrite matrix. The net result is a maximum susceptibility in the region 60-100°C. A number of secondary factors have also been identified, including amount of ferrite, surface condition, presence of cold work and heat tint at welds.
Intergranular Corrosion
Intergranular corrosion is a form of relatively rapid and localised corrosion associated with a defective microstructure known as carbide precipitation. When austenitic steels have been exposed for a period of time in the range of approximately 425 to 850°C, or when the steel has been heated to higher temperatures and allowed to cool through that temperature range at a relatively slow rate (such as occurs after welding or air cooling after annealing), the chromium and carbon in the steel combine to form chromium carbide particles along the grain boundaries throughout the steel. Formation of these carbide particles in the grain boundaries depletes the surrounding metal of chromium and reduces its corrosion resistance, allowing the steel to corrode preferentially along the grain boundaries. Steel in this condition is said to be “sensitised”.
It should be noted that carbide precipitation depends upon carbon content, temperature and time at temperature. The most critical temperature range is around 700°C, at which 0.06% carbon steels will precipitate carbides in about 2 minutes, whereas 0.02% carbon steels are effectively immune from this problem.
It is possible to reclaim steel which suffers from carbide precipitation by heating it above 1000°C, followed by water quenching to retain the carbon and chromium in solution and so prevent the formation of carbides. Most structures which are welded or heated cannot be given this heat treatment and therefore special grades of steel have been designed to avoid this problem. These are the stabilised grades 321 (stabilised with titanium) and 347 (stabilised with niobium). Titanium and niobium each have much higher affinities for carbon than chromium and therefore titanium carbides, niobium carbides and tantalum carbides form instead of chromium carbides, leaving the chromium in solution and ensuring full corrosion resistance.
Another method used to overcome intergranular corrosion is to use the extra low carbon grades such as Grades 316L and304L; these have extremely low carbon levels (generally less than 0.03%) and are therefore considerably more resistant to the precipitation of carbide.
Many environments do not cause intergranular corrosion in sensitised austenitic stainless steels, for example, glacial acetic acid at room temperature, alkaline salt solution such as sodium carbonate, potable water and most inland bodies of fresh water. For such environments, it would not be necessary to be concerned about sensitisation. There is also generally no problem in light gauge steel since it usually cools very quickly following welding or other exposure to high temperatures.
It is also the case that the presence of grain boundary carbides is not harmful to the high temperature strength of stainless steels. Grades which are specifically intended for these applications often intentionally have high carbon contents as this increases their high temperature strength and creep resistance. These are the “H” variants such as grades 304H, 316H, 321Hand 347H, and also 310. All of these have carbon contents deliberately in the range in which precipitation will occur.
Galvanic Corrosion
Because corrosion is an electrochemical process involving the flow of electric current, corrosion can be generated by a galvanic effect which arises from the contact of dissimilar metals in an electrolyte (an electrolyte is an electrically conductive liquid). In fact three conditions are required for galvanic corrosion to proceed; the two metals must be widely separated on the galvanic series (see Figure 2), they must be in electrical contact and their surfaces must be bridged by an electrically conducting fluid. Removal of any of these three conditions will prevent galvanic corrosion.
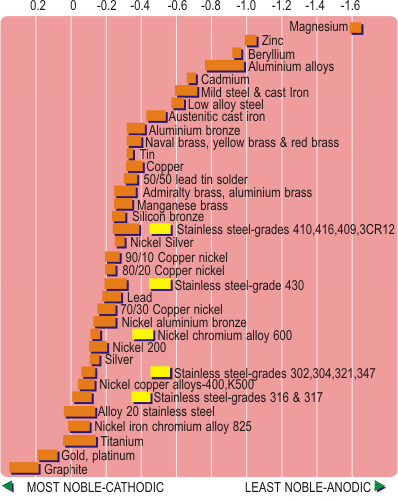
Figure 2. Galvanic series for metals in flowing sea water.
The obvious means of prevention is therefore to avoid mixed metal fabrications. Frequently this is not practical, but prevention can also be by removing the electrical contact – this can be achieved by the use of plastic or rubber washers or sleeves, or by ensuring the absence of the electrolyte such as by improvement to draining or by the use of protective hoods. This effect is also dependent upon the relative areas of the dissimilar metals. If the area of the less noble material (the anodic material, further towards the right in Figure 2) is large compared to that of the more noble (cathodic) the corrosive effect is greatly reduced, and may in fact become negligible. Conversely a large area of noble metal in contact with a small area of less noble will accelerate the galvanic corrosion rate. For example it is common practice to fasten aluminium sheets with stainless steel screws, but aluminium screws in a large area of stainless steel are likely to rapidly corrode.
Contact Corrosion
This combines elements of pitting, crevice and galvanic corrosion, and occurs where small particles of foreign matter, in particular carbon steel, are left on a stainless steel surface. The attack starts as a galvanic cell – the particle of foreign matter is anodic and hence likely to be quickly corroded away, but in severe cases a pit may also form in the stainless steel, and pitting corrosion can continue from this point. The most prevalent cause is debris from nearby grinding of carbon steel, or use of tools contaminated with carbon steel. For this reason some fabricators have dedicated stainless steel workshops where contact with carbon steel is totally avoided.
All workshops and warehouses handling or storing stainless steel tubing must also be aware of this potential problem, and take precautions to prevent it. Protective plastic, wood or carpet strips can be used to prevent contact between stainless steel tube and carbon steel storage racks. Other handling equipment to be protected includes fork lift tynes and crane lifting fixtures. Clean fabric slings have often been found to be a useful alternative.
Passivation and Pickling
If stainless steel does become contaminated by carbon steel debris this can be removed by passivation with dilute nitric acid or pickling with a mix of hydrofluoric and nitric acids.
Source: wilsonpipeline Pipe Industry Co., Limited (www.wilsonpipeline.com)
Comments